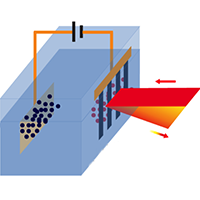
One of the most important things to understand in battery technology is the precise physical and chemical processes that occur at the electrode/electrolyte interface. However, microscopic understanding of these processes is quite limited due to a lack of suitable probing techniques. Now, researchers at the US Department of Energy’s (DOE) Lawrence Berkeley National Laboratory (Berkeley Lab) and the University of California, Berkeley, have developed a new technique that enables sensitive and specific detection of molecules at the electrode/electrolyte interface.
This new method uses diffraction from graphene gratings to overcome key difficulties associated with traditional optical spectroscopy that employs infrared probing of buried interfaces.
“Most of the electrical chemical reaction in a battery happens at the electrolyte/electrode interface, and it is important to know how tuning the electrode voltage induces field-dependent chemical processes. This requires distinction between microscopic molecule behaviour at the interface, such as physical absorption, and electrochemical reaction from the bulky electrolyte solution,” says Feng Wang, a physicist at Berkeley Lab’s Materials Sciences Division and a member of the Kavli Energy NanoSciences Institute at Berkeley, who led this work. “Our new probing method uses diffraction from grating-like graphene electrodes. We monitor the molecule vibration modes from the diffraction signal in an in situ, non-invasive and fast technique, taking advantage of both laser technique and graphene properties.”
Wang is an author of a paper describing this research in Nature Communications. Other authors include Ya-Qing Bie, Jason Horng, Zhiwen Shi, Long Ju, Qin Zhou, and Alex Zettl, who is also a physicist at Berkeley Lab and a member of the Kavli Institute.
“The scientific community now has available impressive techniques for the growth, transfer, and geometrical shaping of graphene for electronic and optical application,” says Zettl. Graphene is an attractive choice of electrode for interface studies because it is stable and transparent to infrared light, and is being explored for applications in supercapacitors, batteries, solar cells and displays.
The novel “diffraction spectroscopy” uses polarised infrared radiation incident to an electrode made of graphene systematically cut into a very fine grid or grating. Together with a platinum counter electrode and aqueous electrolyte, this forms an electrochemical cell. Molecular species within the cell attach to the graphene and thereby influence the diffraction characteristics of the grating.
To investigate the molecular species at the electrolyte/graphene interface, the team measured the first-order diffraction from the graphene grating, rather than the transmission or reflection signal as in traditional spectroscopy.
“We use the fact that the diffraction signal only probes things that have spatially periodic structures, and design our graphene electrodes to be shaped as a periodic grating. In this case, the molecules of interest are periodically distributed due to the underlying electrode grating, and most of the background signals in the traditional reflection or transmission measurement do not show up,” explains Wang.
This means that any measured diffraction originates from vibrations of adsorbed molecules in the graphene-induced electrical double layer. Relative contrast is enhanced 50 times compared with conventional absorption spectroscopy, and can detect with sub-monolayer sensitivity. This proof-of-principle study detected CH2 vibrations from surfactant deposition on the graphene electrode as an example, but the technique can be applied to other functional groups at other infrared frequencies.
“Beyond the vibration range of the methyl groups used in this work, there are plenty of other interesting chemical processes involving molecules whose vibrations are in the infrared range. The more we know about the interface molecule behaviour, the more guidance we have for device design,” concludes Wang.